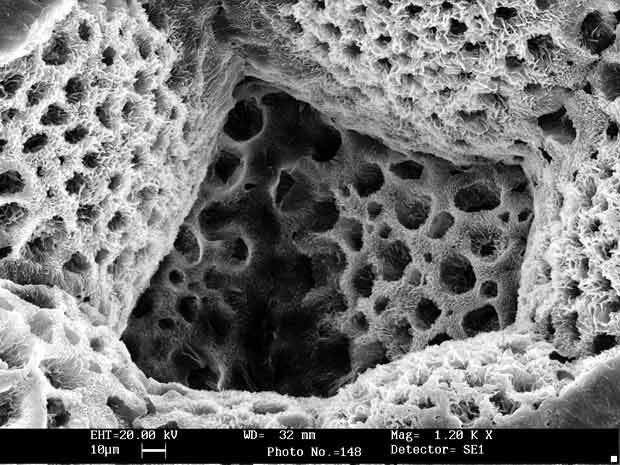
Rapid advances in material science have opened up a new sphere of development in the last decade, and the appetite for development within that field has been voracious. Biomaterials development and tissue engineering have shown enormous potential in many areas of therapy, and as they give up more of their secrets, researchers and manufacturers are keen to identify the specific areas in which they can deliver greatest benefit.
Already some key target areas have been defined. For instance, tissue-derived biomaterials are now often used as xenotransplant materials in the heart valve and vascular prosthesis, in part due to their excellent biocompatibility.
Tissue engineering, combining genetic engineering of cells with chemical engineering to create artificial organs and tissues, has already been applied to skin, bone, heart valve and cartilage for joints. The use of purified porcine islet cells for insulin production and transplant into humans has, for instance, been enhanced by encapsulating them in a synthetic membrane that protects them from rejection by the immune system.
As well as reducing the need for immunosuppression, tissue engineering also has the advantages of minimising the complications associated with traditional transplants. Crucially, it also has the potential to significantly reduce the need for donor tissue, and reduces the presence of foreign material in the body.
“The progress has been tremendous, particularly in the way that in the last ten to 15 years there has been a big collaborative effort from people in many fields, from material science to molecular biology, which helps a lot with development,” says Professor Luigi Ambrosio of the Institute of Composite and Biomedical Materials and the Interdisciplinary Resource Centre on Biomaterials at the University of Naples. “We have been able to clarify many areas of research as well as many material responses. This has contributed to a huge improvement in the use of biomaterials.”
There is no doubt that the pace of development in this area has, so far, been tied to the growing sophistication of the materials available for insertion into the human body. “Before, there were only metal materials available for implant or prosthesis, but now there are many new materials, like polymers and composites,” says Ambrosio. “Composites especially will be used, as they can mimic nature and the body’s movements well and have a close correlation with the surrounding tissue.”
How well do you really know your competitors?
Access the most comprehensive Company Profiles on the market, powered by GlobalData. Save hours of research. Gain competitive edge.
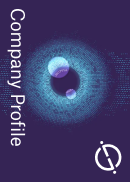
Thank you!
Your download email will arrive shortly
Not ready to buy yet? Download a free sample
We are confident about the unique quality of our Company Profiles. However, we want you to make the most beneficial decision for your business, so we offer a free sample that you can download by submitting the below form
By GlobalDataHowever, the properties of biomaterials themselves do not completely define the focus of development in this sector of research. Of equal importance is the examination of how these materials perform in context. It is here that the cooperation between experts in different disciplines has had the greatest traction, allowing teams to develop workable solutions in far shorter times.
“Now the rapid development is not coming from the materials, but because of issues like interaction, looking at the cells around the materials rather than the material itself,” he remarks. “This is really improving our understanding and this is partly due to collaborative efforts in a complex field.”
CHOOSING THE TARGETS
Among the many areas in which biomaterials and tissue engineering hold great promise, cardiology currently ranks high. Development continues of synthetic polymer-derived polyurethane surfaces with improved biocompatibility, to allow cell attachment for the growth of heart valves and wound-healing applications. Elsewhere, development efforts are focusing on producing ‘off-the-shelf’ ranges of synthetic grafts incorporating multiple biomaterials for coronary and arterial bypass applications.
Another key area in which biomaterials offer much potential is site-specific delivery. Biomaterials have been found to be particularly effective in enabling the controlled release of biologically active agents such as growth factors, as well as for pharmaceutical treatments.
Work continues to further define the release characteristics of different materials and to investigate their applicability to evolving therapies. The success of gene therapy, as well as DNA and anti-sense oligonucleotide delivery for the treatment of many conditions could depend upon their effective, site-specific delivery.
In the Cardiovascular Special Interest Group at Clemson University, for instance, current research is focusing on the development of a hydrogel system, which could be used to deliver plasmid DNA and adenovirus containing DNA to specific sites for applications such as would healing, restenosis and calcification. So far, research indicates that such a system can slowly release adenovirus particles in active form either in cell structures or in animal models, transfecting cells for several days.
As well as this type of application, pharmacological agents can also be attached to polymers such as collagen, glycosaminoglycans or synthetic agents like acrylate/methacrylates. These can be released when trigger mechanisms, such as a higher local expression of enzymes, come into play.
INDUSTRY ADVANCES
In 2005, Affinergy, a spin-off from Duke University, was awarded the Frost & Sullivan Technology Innovation of the Year Award for medical coatings/biocoatings technologies, for its site-specific biological delivery technology interfacial biomaterials (IFBM). IFBMs are modular structures that include two functional peptides, one designed to selectively bind to a specific biological material, while the other selectively binds to a specific target surface, be it biological or synthetic.
The two peptides are joined through a proprietary linker technology, which offers advanced capabilities such as cleavability or cross-linking. Affinergy is now building and patenting a ‘library’ of peptides that bind with high affinity and high selectivity to the bioactive and synthetic materials widely used in the biomedical and therapeutic industries.
The company is also working on further developments to its site-specific delivery system, which it intends to validate for improved cell attachment to metal implant surfaces typically used in areas such as orthopaedic implants, cartilage repair, and growth factor delivery.
A further area in which there is great potential for biomaterials to improve the range of available treatments is in the orthopaedic area. Many bone graft substitutes are in development, made from collagen, polymers or composite biomaterials.
Kensey Nash Corporation, for instance, has been engaged in late-stage clinical trials of an in vivo three-dimensional implant for articular cartilage repair and regeneration. It has developed an implant from numerous biomaterials that is architecturally stratified to accommodate the different components of articular cartilage, including the underlying subchondral bone region.
At the University of Naples, Ambrosio has been working on a number of projects, but principally artificial disc prosthesis and the generation of the meniscus system for cell attachment. More innovative still is his focus on the regeneration of tissue in the meniscus, which ultimately would eliminate the need for its removal.
“From my point of view, I am trying to regenerate pathological tissue. That means regenerating healthy tissue, not just replacing it. That is the challenge, and it is a difficult one. Sometimes the cells in the tissue we are trying to regenerate are already dead or function badly.”
PUSHING THE MARKET OPPORTUNITY
The cost of research into tissue engineering and biomaterials is a cause of concern, but Ambrosio recognises that this is true of any area of medical device development.
“Of course for us scientists the funding is never enough,” he remarks. “By the time funding comes through, costs have risen. It is a critical issue.”
Given the rapid pace of change in biomaterials applications and tissue engineering capabilities, the commercial opportunities are certainly there, and this is likely to attract growing levels of investment from major manufacturers. If solutions can be found to treat common problems, particularly those that involve limitation of physical capability, then demand for solutions will be high.
Progress has been made, as mentioned above, in the treatment of diabetes, which affects a large and growing patient population worldwide. Ambrosio sees similar potential in the treatment of spine problems.
“In simple orthopaedics, for instance, our spine project targets lower back pain, which is a very common pathology and can now start at a very young age,” he says. “We sit more and we work a lot at computers. At the moment, there is no good solution, only temporary medical treatments. This area needs a lot of money invested in it.”
While more funding would certainly help to bring successful products to market faster, throwing money at the challenges would not be enough to optimise such efforts. Ambrosio and others feel that it is time for the many experts from diverse disciplines involved in the development of tissue engineering and biomaterials to pause and take stock.
CLARITY AND PURPOSE
Such has been the rate of advance in materials, applications and expertise over recent years that the framework, and in some cases the terminology, that define the disciplines of biomaterials and tissue engineering have become less clear. Certainly Ambrosio, who has been busy organising a recent conference to bring together the many experts involved in these fields, feels that one issue such minds have to address is how to formalise a new framework for future development.
To highlight this need, he points to a key term – biocompatibility – that has outgrown its previous definition because of the huge leaps that have been made in the last few years. Until now, he points out, tests for biocompatibility have largely focused on determining one essential factor: whether or not a material is toxic. Progress, it seems, now demands that a more sophisticated approach be taken to investigating, understanding and defining biocompatibility.
“We need to know a material is not toxic, but we also need to know how it interacts with the surrounding systems in the body,” believes Ambrosio. “The pace of development has been so fast that the definitions of biocompatibility and other terms that we have used in the past are no longer enough. So, we must redefine them.”
Ambrosio also feels that a greater emphasis on interaction, rather than on materials, will lead technology developers to look at the long-term effects and performance of implanted medical devices made from sophisticated biomaterials and of tissue-derived solutions. It is from this long-term point of view, he believes, that the optimum applications of tissue engineering and biomaterials will become clear.
Focusing on new short-term opportunities to highlight potential applications has opened the field up, but the delivery of solutions that offer tangible benefits to patients will depend upon a clearer understanding of the complex structures that encompass any implanted device or material.
“The body is always changing,” he says. “Its chemical make-up changes and so does its structure. It takes in new pharmaceutical treatments and so on, and materials behave differently in a biomechanical sense. So, we must put the body and the materials together and examine them in the long-term.”
EXPERT COLLABORATION
Within a better-defined framework, Ambrosio believes that the high levels of cooperation between experts across the involved disciplines will continue, with excellent results. Though such collaboration does not guarantee that development processes are optimised, it certainly fuels the interest and enthusiasm that has taken the field so far forward in such a short time.
Now, he feels it is time to collate the knowledge that has been accumulated over diverse research projects to provide a new starting point, some level ground from which to mount the next stage of development, which will yield tangible, practical solutions for many patient populations.
“There are very few new synthesised materials, but they can be used in different combinations”, he says. “So, we must put together all the data we have. There are already so many materials we can use in the body, but we can go a long way by bringing together all the data on those processes. We can’t do it without widespread collaboration.”
Already there are signs that manufacturers are vying for position in the market, and this is fuelling the desire for usable, cutting-edge solutions. “There are many applications – in orthopaedics, cardiovascular treatment, soft tissue replacement – and companies are starting to see that it is an important area,” adds Ambrosio. “There is a lot of competition so they are looking to come to the market with advanced systems.”
With the combined energies of competition and collaboration fuelling their growth, tissue engineering and biomaterials are likely to be the topics on everyone’s lips for a long time to come.