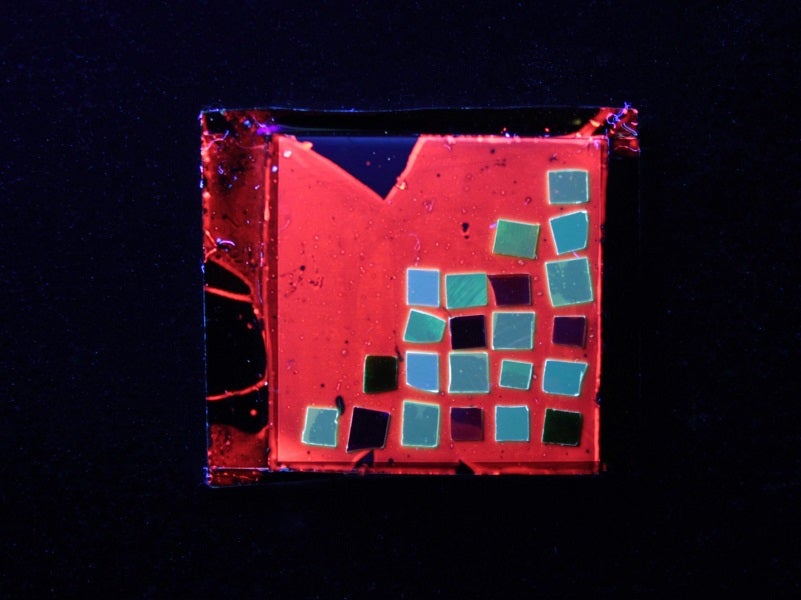
2010: researchers see the atoms of a virus for the first time
Researchers at University College Los Angeles (UCLA) were able to image the structure of a virus at such a high resolution they were able to see its individual atoms, in the first published incidence of imaging biological matter at such a resolution.
The researchers were able to image the viruses at 3.3 angstroms. An angstrom is the smallest recognised division of a chemical element, around the distance between the two hydrogen atoms in a water molecule.
This was done using cryogenic electron microscopy (cryo-EM), an electron microscopy technique applied on samples that have been cooled to cryogenic temperatures and embedded in amorphous ice. Electron microscopy uses a beam of accelerated electrons as a source of illumination, passing through empty areas and bouncing off dense ones. A digital camera then reads the path of the electrons passing through the sample to create a 2D projection image of the sample, which a computer can then use to construct a three-dimensional image as the process is repeated at hundreds of different angles.
Viruses can be classed as either enveloped or non-enveloped, based on whether they are surrounded by a membrane that fuses with and infects cells, or whether they do so using a protein instead. The virus looked at in this study, the aquareovirus, is a non-envelope type that causes disease in fish and shellfish. Using the hi-res cyro-EM images, the researchers were able to determine that the virus employs a priming stage to accomplish cell infection. In its dormant state, the virus has a protective protein covering, which it sheds before using a protein called an “insertion finger” to infect a cell.
2014: Nobel Prize awarded to researchers who surpassed the limitations of the light microscope
Two Americans and a German, all working separately, jointly received the 2014 Nobel Prize in chemistry for their work on optical microscopy.
Optical microscopy had previously been held back by a presumed limitation that it would never obtain a better resolution than half the wavelength of light. However, the research projects completed by Stefan Hell, William Moerner and Eric Betzig were able to bring optical microscopy into the nanodimension.
How well do you really know your competitors?
Access the most comprehensive Company Profiles on the market, powered by GlobalData. Save hours of research. Gain competitive edge.
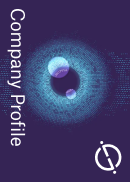
Thank you!
Your download email will arrive shortly
Not ready to buy yet? Download a free sample
We are confident about the unique quality of our Company Profiles. However, we want you to make the most beneficial decision for your business, so we offer a free sample that you can download by submitting the below form
By GlobalDataHell’s stimulated emission depletion (STED) microscopy was developed in 2000. The technique utilises two laser beans, one stimulates fluorescent molecules to glow and the other cancels out all fluorescence bar that at a nanometre-sized volume. Scanning over a sample nanometre by nanometre can yield an image resolution better than the stipulated limit of half the wavelength of light.
Meanwhile, Betzig and Moerner were separately laying the foundation for single-molecule microscopy. Relying on the possibility to turn the fluorescence of individual molecules off and on again, the same area is imaged multiple times with just a few interspersed molecules allowed to glow each time. Superimposing the images yields a dense super-image resolved at the nanolevel.
Since their inventions, nanoscopy has allowed researchers to visualise the pathways inside living cells. They have been able to see how synapses move between nerve cells in the brain, track the proteins involved in neurodegenerative diseases and follow proteins in fertilised eggs as they divide into embryos.
2017: mobile microscopes abound
A surge of mobile phone microscope innovation took place in 2017, as researchers across the US and Europe developed devices that use a smartphone camera to take a closer look at things.
In January 2017, researchers from University College Los Angeles (UCLA) and Uppsala University developed a small 3D-printed microscope, which fits to a mobile phone and assists in the DNA-sequencing of tumour cells and tissue samples. A sample is simply placed in a small container, and the mobile phone microscope records multi-mode images of the process sample into an algorithm, which automatically examines the images to read the sequenced DNA bases.
The microscope, which can capture multi-colour fluorescence and bright-field images, identifies the presence of targeted DNA sequences while examining the samples for tumours, bacteria, virus or fungal cells.
2019: infrared laser beams for microscopic melanomas
At the University of British Columbia researchers were able to develop a laser microscope with the potential to diagnose diseases like skin cancer and perform precision surgery without a single cut to the skin.
The device is a specialised multiphoton excitation microscope that images living tissue up to one millimetre in depth using an ultrafast infrared laser beam. The microscope goes beyond simply scanning living tissue, instead, it treats it by intensifying the heat produced by the laser.
When applied to skin diseases like melanomas, the microscope allows medical professionals to pinpoint the exact location of the abnormality, before diagnosing and treating it instantly. It could theoretically be used to treat any structure in the body reached by light, including nerves or blood vessels in the skin, eyes or brain.
The research team behind the device are now exploring whether a mini version of the microscope could be developed to perform microscopic examinations and treatment during endoscopic procedures.
2020: dark-field quantum dots
Dark-field microscopy involves placing brightly lit samples against a black background to gain a clearer view of a sample, with better contrast.
Engineers at the Massachusetts Institute of Technology have developed a small, mirrored chip that helps to produce dark-field microscopy images without the need for dedicated expensive components. Slightly larger than a postage stamp and as thin as a credit card, the chip emits a hollow cone of light that can be used to generate detailed dark-field images of bacteria, as well as algae and similarly translucent tiny objects.
The chip is designed to be added to standard microscopes as an affordable alternative to conventional dark-field equipment.
The research team tested the chip using seawater and non-pathogenic strains of E. coli, and placed each sample on the chip on the platform of a standard bright-field microscope. They were able to produce clear, detailed images of individual microorganisms and bacterial cells, which were close to invisible under bright-field illumination.