
Image-guided intervention (IGI) robots have stringent requirements for imager compatibility, precision, sterility and safety, as well as size and ergonomics. A robot’s compatibility with a medical imager refers to the capability of the robot to safely operate within the confined space of the imager while performing its clinical function, without interfering with imager functionality. Among all types of imagers, the MRI is the most demanding and the development of MRI robots is a challenging engineering task.
MRI scanners use magnetic fields of extremely high density (such as 3 Tesla), with pulsed magnetic and radio frequency fields. Ferromagnetic materials are exposed to high magnetic interaction forces and heating may occur in conductive materials by electro-magnetic induction. Electricity use may cause interference, leading to signal to noise attenuation, signal distortions and image artifacts. Most of the components used in robotics may not be used in close proximity of the MRI. The most common type of motor used in robots is electromagnetic, clearly incompatible with the MRI.
EARLIER MOTOR USE: PIEZOELECTRIC
Previous research has used piezoelectric motors, also called ultrasonic motors. The piezoelectric effect is the ability of some crystals and certain ceramic materials to generate a voltage in response to applied mechanical stress. The effect is reversible, so in motors piezoelectric crystals change shape and produce motion when subjected to an externally applied voltage.
The problem with piezo effect is that the change in the shape of the piezo crystal is very small (~0.1%) and this is only achieved under high voltages. In order to create usable motion, the voltage needs to be pulsed at high frequencies. These violate the patient safety and imager non-interference requirements of compatibility.
Piezo motors have slightly different performance and characteristics, but these create distortions if operated closer than 0.5m from the image isocentre. Researchers dealt with this by deactivating the robot during imaging. But even without power, the wiring of the motors still takes a significant part of the MRI signal, typically debasing the signal-to-noise ratio by as much as 50%. Employing complicated electric screening and filtering solutions resulted only in minimal gain.
How well do you really know your competitors?
Access the most comprehensive Company Profiles on the market, powered by GlobalData. Save hours of research. Gain competitive edge.
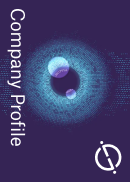
Thank you!
Your download email will arrive shortly
Not ready to buy yet? Download a free sample
We are confident about the unique quality of our Company Profiles. However, we want you to make the most beneficial decision for your business, so we offer a free sample that you can download by submitting the below form
By GlobalDataA recent study addressed the compatibility of a new piezoelectric motor made of ceramics (SQUIGGLE motor, New Scale Technologies, Rochester, NY, US) in a 4.7T animal research scanner. Unfortunately, image distortion tests were not performed but the study mentioned that the motor has to be kept distal and additional electronic filtering work is in progress.
The piezoelectric effect is magnetism free; however, piezo motors are not MRI compatible. Piezo motors operate on patient unsafe voltages and interfere with the functionality of the imager. MRI sequences may not be selected based on imaging requirements but are limited by compatibility. Piezo actuation is a compromise used in the absence of a better solution.
Few other actuators were used. A group from Switzerland has recently reported developments on using hydraulic actuation, and is advocating the advantages of hydraulic master-slave coupling for MRI actuation. Hydraulic actuators could be nonmagnetic and dielectric for full MRI compatibility. The group has also reported development on optical force sensors to be used in feedback. These are promising solutions, if leakage could be controlled. Hydraulic actuators are notorious for fluid loss, a
major sterility concern.
IDEAL MATERIALS AND ENERGY
Several non-ferrous metals such as titanium and nitinol have been found to be acceptable for small parts and are being used in commercial MRI instrumentation. However, for noninterference with electromagnetism the ideal materials should be nonmagnetic but also dielectric. There are plastics, ceramics, rubbers and glasses.
From the energetic point of view, electricity is not MRI compatible because currents generate electromagnetic waves and require conductor materials in which electricity is being induced from the scanner. Pneumatics and light on the other hand are ideal choices, because these are decoupled from electromagnetism.
PNEUMATIC MOTORS
Pneumatic actuation is a fundamentally flawless option for MRI compatibility. The Institute for Medical Engineering and Biophysics (IMB), Karlsruhe, Germany was the first to realise this after failed attempts with piezo actuation. The institute’s last version used a cylinder to drive an end-effector axis. The institute is no longer active, but spin-off company Innomedic is developing a pneumatic robot for CT or MRI-guided needle procedures.
The major limitation of pneumatic actuators has been their reduced precision in controlled motion. Pneumatics is traditionally used for free-spinning motion such as drills or in industrial automation such as opening and closing gates. Pneumatic motors (turbine or cylinder based) are fast and powerful but are notoriously hard to control if the motion is to be precisely regulated as needed for robots.
In MRI pneumatic servo control is even more intricate because the electric control valves need to be distally located and long connection hoses are needed. The compressibility of the air in the long hoses coupled with the friction of the actuator and valves makes the system highly nonlinear, hardly manageable and susceptible to small disturbances, and it raises significant safety concerns. A system currently under development considers the use of piezoelectric valves, which would allow for placing the valves closer to the scanner to shorten the hoses, simplifying the control.
The main safety concern for using cylinders in medical applications is that these are direct drive actuators. If malfunctioning, direct drive systems may swiftly spring off, quickly unwinding and potentially hurting patients/personnel.
Medical applications require small, slow, precise and safe actuation. No classic pneumatic motor could satisfy the reliability, precision and safety required for a medical robot.
COMPATIBLE NEW TECHNOLOGY
Rather than coping with the piezo motor MRI incompatibilities, trying to control the existing types of pneumatics and avoid known engineering problems, a new type of motor specifically designed for this application was created by the URobotics team at John Hopkins Medicine in Baltimore, US. PneuStep uses a step motor principle to achieve precise motion in a safe and easily controllable manner. It is the first and only ‘MRI Stealth’ motor.
The PneuStep invention is based on the idea that ‘end-to-end motion of a piston within its cylinder is always exact’. This can be achieved by pressurising the cylinder, easier than positioning the piston in midstroke with pneumatic servo-control. The step motor is designed to successively collect small end-to-end motion strokes in a rotary motion. A step is made by an end-of-stroke motion.
The motor is driven by three diaphragm cylinders. The pressure acting on the diaphragms sets a triple parallelogram mechanism in motion and an internal gear in a translational motion on a circular trajectory. This then engages a central spur gear, which is the geared output of the motor. Step motion is achieved by sequentially pressurising the diaphragms. Direction is given by the order of the sequence. The three ports of the motor are connected to a pneumatic distributor for generating the commutation pressure waves. The pneumatic distributor was constructed using three electric valves mounted on a manifold.
Optical encoding was added to the motor to monitor or control its motion. For MRI compatibility, fibre-optic encoding was used, keeping the motor electricity free. An electronic driver controls the new motor with electric stepper indexers and standard motion control cards. The motor accepts open-loop step operation as well as closed-loop control with position feedback from the enclosed sensor.
MRBOT: MRI-GUIDED ACCESS
Six PneuStep motors were used to actuate the first fully actuated MRI-compatible robot, MrBot. Previously reported MRI robots had limited compatibility, mainly due to their piezoelectric actuation. MrBot is customised for the prostate and operates transperineally for needle interventions under direct MRI guidance. It can accommodate various needle drivers for different percutaneous procedures such as biopsy, thermal ablations or brachytherapy. Its first application is for prostate low–dose radiation seed brachytherapy.
PneuStep performance matches the requirements of the clinical application for low speed (<20mm/s), high accuracy (<0.5mm), and most importantly safety. The stepper is safer than servo-pneumatic actuation, because in case of malfunction it may only stall. Breaking a PneuStep hose, for example, may not unwind the mechanism, potentially harming the patient.
The robot is controlled from a remotely located cabinet through 7m hoses carrying air and fibre optics. The robot is entirely nonmagnetic and dielectric. Imager compatibility tests performed showed that the robot is unperceivable in MRI and does not interfere with the functionality of the imager, in motion or at rest. In fact, the robot is multi-imager compatible, because it is compatible with all other types of medical imaging equipment (MRI gives the most stringent constraints).
Motion tests showed the mean value of the robot’s positioning repeatability to be 0.076mm with a standard deviation of 0.035mm, which is impressive for a ‘plastic’ robot. The PneuStep motor was also tested in a 7T MRI scanner, and no problems were encountered in its operation. Mockup, cadaver and animal tests are in progress in reparation for clinical trials.
Image-guided robots not only augment physicians’ manipulation capabilities but establish a digital platform for integrating medical imaging data. This gives robots abilities unattainable to humans, because, unlike humans, robots and imagers are digital devices. But new technologies are in demand for these applications, especially for MRI guidance. The PneuStep is the first motor that can safely, precisely and reliably operate in the MRI without interfering with the image. This is an enabling technology for future MRI instrumentation.